Viral safety in viral vector applications – what you need to know?
Viral contaminations are rare events in biopharmaceutical manufacturing, but regulatory agencies expect all biologics to undergo viral clearance testing to mitigate the risk of contamination [1, 2]. Therapies based on viral vectors have a small but evident potential for viral contamination. The unique challenge arises from the physicochemical similarity between the gene therapy product and the adventitious viral counterparts. When the product itself is a virus, how will potential viral contaminants be eliminated? A detailed understanding of subtle differences is required, as well as a good knowledge of technologies or processes that can efficiently remove impurities from the product. Here we review some of the concepts of viral risk mitigation strategies in the manufacturing of viral vector-based gene therapy products.
1. What are the sources of viral contamination?
There are numerous routes through which adventitious viruses may be introduced into the viral vector manufacturing process. A study published in Nature Biotechnology offers insight into the most common sources, namely 1) raw materials, 2) cell cultures and 3) open operations [3].
Most viral contaminations related to raw materials are associated with the use of animal-derived materials. Materials such as serum, trypsin, plasma proteins, and tissue and protein extracts can potentially contain viral contaminants. Of non-animal raw materials, glucose carries the highest risk for viral contamination in the upstream process, if a chemically defined medium is used.
Viral contaminants in the producer cell lines can originate from the cell source, from the materials used in cell culture, or from exposure to the operator or environment. Cells may have a latent or persistent virus infection (e.g. herpes virus or endogenous retrovirus). Open manufacturing operations such as open cell-culture transfers can expose the process to viral contamination from the operators or other environmental factors.
2. Risk mitigation strategies
The mitigation plans of the manufacturers follow three complementary approaches: prevent, test, and remove/inactivate. In addition, viral clearance studies can be used to check the production process for the efficacy of removing adventitious viruses.
Prevent – Raw material risk mitigation
Raw material de-risking eliminates or reduces the viral contamination load through complementary approaches. In addition to selecting low-risk vendors, the manufacturers can use pretreated products such as High-Temperature Short Time (HTST) pretreated glucose. The use of defined media that do not include raw materials of animal or human origin reduces the risk of upstream viral contamination and is often the preferred approach. Also, virus retentive filtration can be used for chemically defined cell culture media. It is worth noting that data submitted to the Consortium on Adventitious Agent Contamination in Biomanufacturing (CAACB) demonstrated that the control of raw materials by direct testing had limited value. In the reported cases initial testing of raw materials did not detect the viruses. On some occasions, the virus was detected in the raw material, but only after increasing the viral load through concentration or biological amplification [3]. New technologies, such as massively parallel sequencing, may improve the identification of the viral contaminants present in raw materials and help in implementing screening programs to assess raw material safety [4].
Test -Cell and virus banks and bulk harvest
According to EMA and FDA guidelines, testing for adventitious viral agents should be implemented for cell banks, virus seed stocks, control cells, and bulk harvests [1, 2]. If plasmids are used in viral vector manufacturing, only microbial cell banks free from viruses should be used. Control cell testing for adventitious viruses is only required at those time points when the tests are performed on the manufactured viral vector product. Examples of relevant tests for adventitious agents at various production stages are listed in European Pharmacopoeia (Ph. Eur.) 2.6.16.
Part of the risk mitigation is to avoid cell culture contamination by operators. The reduction in contamination probability can be achieved by using closed systems and single-use disposable materials. If open operations cannot be avoided, those steps must be performed in biosafety cabinets with HEPA-filtered air. Operators must also be appropriately gowned and trained for aseptic work.
Remove/inactivate – Viral clearance
Viral clearance is a key regulatory requirement for all recombinant biopharmaceuticals and is outlined in the ICH Q5A guideline. Typical viral clearance strategies for e.g. AAV products use a combination of filtration, inactivation, and chromatographic steps. The unit operations depend on various factors and should be considered case-by-case and step-by-step since different viruses have different biophysical characteristics. Factors that impact the clearance are the lipid envelope (enveloped or non-enveloped virus), particle size (small or large virus), genome (DNA or RNA, small or large genome), and surface charge (negative or positive charge under specific buffer conditions). However, the viral clearance choices using viral inactivation and filtration may be limited due to the danger of influence on the product itself. Therefore chromatographic separation steps, especially affinity-based, are important in the efficient elimination of adventitious viruses.
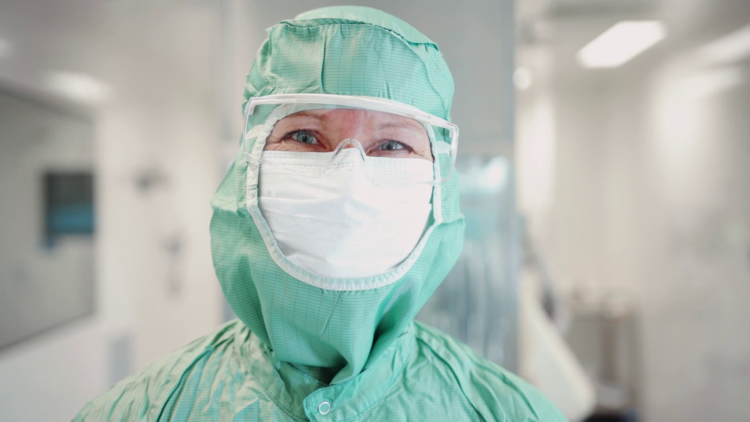
3. Viral clearance studies
Viral clearance studies involve spiking viruses into process intermediates and then demonstrating their inactivation or removal during the subsequent processing steps. According to EMA’s Committee for Medicinal Products for Human Use (CHMP), it is desirable to investigate the contribution of more than one production step to viral clearance, and at least two orthogonal steps should be considered. Orthogonal steps are process steps where different mechanisms are used for virus inactivation or removal [5]. It is worth noting that viral clearance studies are usually done using a scaled-down version and in an external laboratory by staff with virological expertise.
The selection of viruses for viral clearance studies
The manufacturer should explain the rationale for the choice of viruses in the aims of the study. Viruses for clearance evaluation and process characterization studies should be chosen to resemble viruses that may contaminate the product. They should also represent a wide range of physicochemical properties to test the ability of the system to eliminate viruses in general. According to the ICH QA5 guideline, the viral clearance studies should use ‘relevant’ viruses, ‘specific model’ viruses, and ‘non-specific model’ viruses. A relevant virus is an identified virus of the same species as the virus that is known to, or likely to, contaminate reagents or materials. A specific model virus is closely related to a known or suspected virus (same family genus). A non-specific model virus is a virus with different properties for characterizing the capacity of the manufacturing process to remove or inactivate viruses in general [2]. It should be possible to grow the viruses selected for viral clearance studies to high titer and there should be an efficient and reliable assay for detecting each virus at every stage of manufacturing that is tested. One should consider the health hazards to the personnel performing the clearance studies (e.g. avoid BSL3 and BSL4 class viruses). According to CHMP’s note for guidance, if two similar viruses could be used for viral clearance studies either because of their equal resemblance to possible contaminants or similarities in their properties, the virus considered more resistant should be used [6].
Viral clearance study for AAV –an example
The ICH Q5A is currently under revision (expected final version end of 2023); the new version will provide detailed guidance and recommendation on viral clearance studies for gene therapy vectors. Until then the choice of viruses for the clearance study for AAV-based products has to be based on the risk assessment of the raw materials and processes, experience from other products, such as recombinant proteins, as well as scientific reasoning. In addition, the FDA CMC for gene therapy INDs (2020) states “In some instances, robust viral clearance studies may be necessary to remove and inactivate adventitious agents”.
AAV is a highly resistant, non-enveloped virus, and solvent/detergent treatment steps were in tests found to have limited effect on the AAV vector. However, the treatment will inactivate enveloped viruses such as retroviruses and herpesviruses. Reduction by filtration could be performed using 35-50nm pore size filters that remove larger enveloped and non-enveloped viruses but allow AAV (~25nm) to pass into the filtrate. This approach could remove and inactivate enveloped viruses of all sizes and non-enveloped viruses >35-50nm.
Chromatographic steps used for purification and polishing can be combinations of affinity, ion exchange, hydrophobic interaction, and size exclusion. For example, affinity chromatography and anion exchange chromatography contribute to viral reduction and can be applied to both enveloped and non-enveloped viruses. Especially affinity resins that specifically bind products, combined with adequate washing regimes, can effectively eliminate adventitious viruses. The resin-based approaches are widely used in commercial manufacturing, but new technologies such as membrane chromatography and monolith chromatography have emerged. They can potentially provide additional viral removal and increase product purity.
One approach adopted for products derived from CHO and rodent cells is to use a ‘worst case’ virus (having the strongest physicochemical resemblance to the product). The AAV manufacturing process based on Hek293 cells could be spiked with a xenotropic murine leukemia virus, XMuLV (80-120 nm, highly charged, enveloped ssRNA virus), or pseudorabies virus, PRV (~ 200 nm, enveloped dsDNA virus). A good model for validating robust filtration solutions is also a moderate size reovirus type 3, Reo-3 (~ 70 nm, non-enveloped virus). This selection would cover different sizes and physiochemical properties of adventitious viruses. The rationale behind this selection would be that PRV and Reo3 may be introduced through serum or trypsin (if used), whereas XMuLV can originate from infected rodents that have contaminated glucose. Since a human-derived cell line, HEK293, is used, human hepatitis A, HAV (~27-32nm, ssRNA, non-enveloped virus) and herpes simplex virus 1, HSV-1, (~ 155-200nm, dsDNA, enveloped virus) could be tested. It is worth mentioning that regulatory authorities accept only data from viral clearance studies obtained with live viruses. Consequently, noninfectious viral surrogates such as Mock-V preparation can only be used for process development and optimization of viral clearance studies.
The remaining challenge
The remaining challenge with AAV viral clearance is other parvoviruses, such as Porcine parvovirus, PPV (~18-24nm, non-enveloped), or Minute virus of mice, MVM (~14nm, non-enveloped). They are very similar in size to the AAV-based products and cannot be removed by the filtration process. In addition, they will not be inactivated by treatment with low pH or solvent/detergent. Subsequent chromatographic purification may provide reduction, but if the charge of the spiked virus is similar to the AAV vector, they are likely to co-elute. Because parvovirus contamination may originate from the use of glucose in the process, due to the potential of rodents in the warehouse, regular glucose testing before use, as well as a well-controlled raw material supply, are crucial for the viral clearance risk assessment. In addition, it should be obligatory to screen starting materials and raw materials with new molecular methods, such as quantitative PCR or next-generation sequencing (NGS), when viral clearance is difficult or impossible [7].
Summary
Viral vector-based products must be free of viral contaminants. Because of the similarities between the product and adventitious viruses, the manufacturers must know the sources of contaminations and establish specific processes to remove them. Viral clearance studies provide hard data ensuring the biosafety for human use of the new products. The new revision of EMA Q5A will provide more guidance and recommendations on ensuring viral safety during the manufacturing of gene therapy vectors.
- FDA Guidance for Industry, Chemistry, Manufacturing, and Control (CMC) Information for Human Gene Therapy Investigational New Drug Applications (INDs)
- EMA ICH, Q5A Guideline on virus safety evaluation of biotechnological investigational medicinal products.
- Nature 38, 563–572 (2020) Viral contamination in biologic manufacture and implications for emerging therapies.
- Onions D, Kolman J. (2010) Massively parallel sequencing, a new method for detecting adventitious agents. Biologicals.38:p377-380
- EMEA/CHMP/BWP/398498/2005, Virus safety evaluation of biotechnological investigational medicinal products
- EMEA/CHMP/1996/note for guidance, Virus validation studies: the design, contribution, and interpretation of studies validating the inactivation and removal of viruses
- Next-Generation Sequencing: The Utilization and Challenges for the Advancement of Viral Detection in the Biopharmaceutical Industry.